Silicon detector array
Our goal is to construct an array of double-sided silicon strip detectors (DSSDs), comprising 8 DSSDs with front-end electronics ASIC readout, to greatly improve the detection system used in our previous reaction and beta-decay experiments (Figure 1). The newly developed ASIC on market for nuclear physics instrumentation is “GET” (stands for General Electronics for TPCs), which is a generic, reconfigurable and comprehensive electronics and data acquisition system for instrumentation of up to 33792 channels. As in Ref [1,2], the GET system consists of a custom-designed ASIC for signal processing, 256-channel front-end cards that each houses 4 ASIC chips and digitize the data in parallel through ADCs, concentration boards to read and process the digital data from up to 16 ASICs controlled by the associated firmware, communication and data-acquisition software.
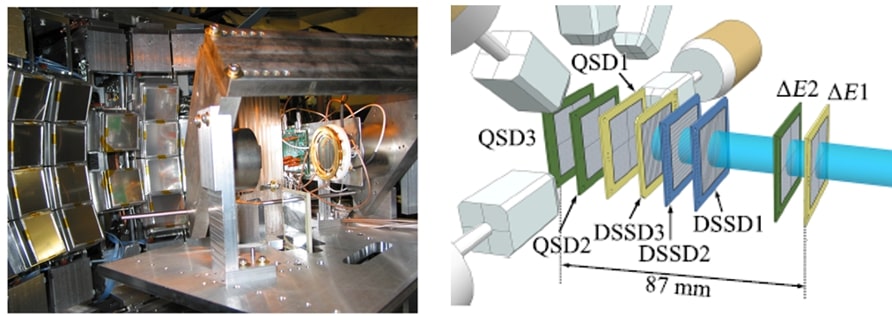
A Si-GET test bench is built in our laboratory with GET readout electronics and vacuum chamber as shown in Figure 2. A DSSD was placed inside the vacuum chamber to detect the emitted alpha particles with energies of 5157 keV, 5486 keV and 5805 keV from a triple-alpha radioactive source. The DSSD was connected to the GET readout outside the chamber via a converter board Si-ZAP designed by our group. Capacitor with three different capacitance of 1nf, 10nf and 300pf were tested, and 1nf was chosen because of the best impedance matching and performance. Cobo and MuTant together with MCH crate and Narval DAQ were used for the full-readout setup. We followed the algorithm in Ref. [3] to analyze data with proper background-noise subtraction. Figure 3 demonstrates the summary spectrum of a DSSD with 128 channels. Channels without full spectrum were strips located at the edge of DSSD and alpha particles were blocked by mapping mask Figure 4 (left) and 4 (right) shows the 1-D energy spectrum in one strip with the GET gain value of 240fC and 1pC respectively which correspond to 5.5 MeV and 20MeV dynamic range. Excellent energy resolutions of 0.65% and 0.93% (FWHM) for 5157 keV alpha particles were obtained, which is in general better than the conventional electronics of 1%. Coincidence between the front and back strips as well as adjacent strips were also checked. In addition, a mask with “HKU” mark was covered in front of DSSD, the resulting hit pattern as shown in figure 5 validates the readout mapping. The satisfactory result of performance test at HKU indicates good applicability of GET readout electronics to the DSSD.
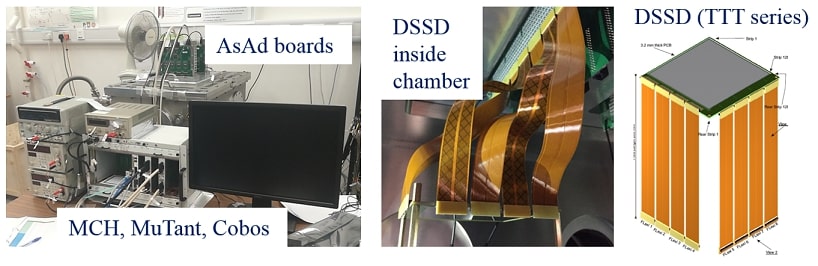
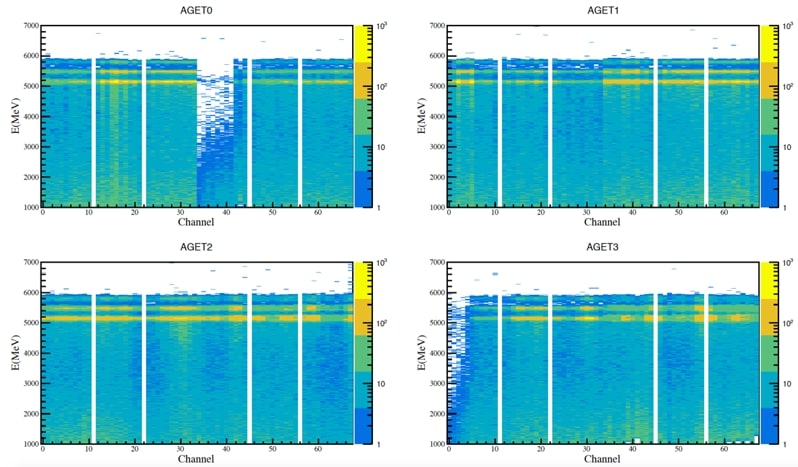
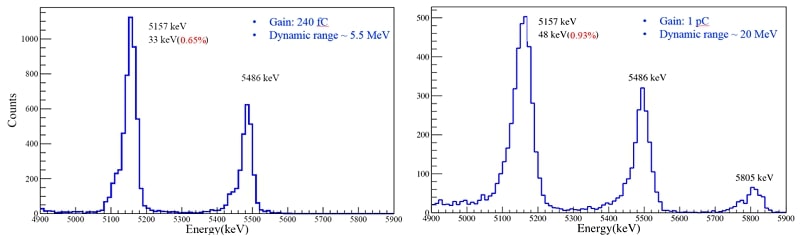
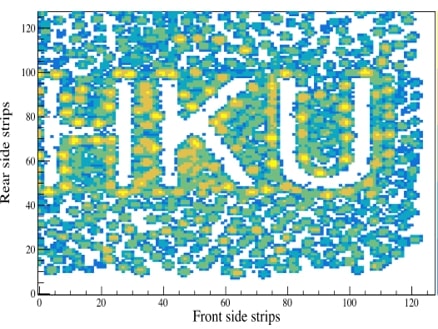
The next step is to further examine the entire system Si+GET in the complex experimental conditions with beams. In particular, we plan to test the stability of the entire system for long-hour data taking, noise pickup and influence, energy resolutions, trigger conditions and limit of counting rate, Narval DAQ integration with other DAQ systems etc. Therefore, we will perform a commissioning experiment using 4 DSSDs with 1024 readout channels at the facility HIRFL-RIBLL in Institute of Modern Physics (IMP) in 2019.
- Nuclear Inst. and Methods in Physics Research, A 887, 81 (2018)
E.C. Pollacco, G.F. Grinyer, F. Abu-Nimeh, T. Ahn, S. Anvar, A. Arokiaraj, Y. Ayyad, H. Baba, M. Babo, P. Baron, D. Bazin, S. Beceiro-Novo, C. Belkhiria, M. Blaizot, B. Blank, J. Bradt, G. Cardella, L. Carpenter, S. Ceruti, E. De Filippo, E. Delagnes, S. De Luca, H. De Witte, F. Druillole, B. Duclos, F. Favela, A. Fritsch, J. Giovinazzo, C. Gueye, T. Isobe, P. Hellmuth, C. Huss, B. Lachacinski, A.T. Laffoley, G. Lebertre, L. Legeard, W.G. Lynch, T. Marchi, L. Martina, C. Maugeais, W. Mittig, L. Nalpas, E.V. Pagano, J. Pancin, O. Poleshchuk, J.L. Pedroza, J. Pibernat, S. Primault, R. Raabe, B. Raine, A. Rebii, M. Renaud, T. Roger, P. Roussel-Chomaz, P. Russotto, G. Saccà, F. Saillant, P. Sizun, D. Suzuki, J.A. Swartz, A. Tizon, N. Usher, G. Wittwer, J.C. Yang
- Eur. Phys. J. A 50: 8 (2014)
A. Obertelli, A. Delbart, S. Anvar, L. Audirac, G. Authelet, H. Baba, B. Bruyneel, D. Calvet, F. Chˆateau, A. Corsi1, P. Doornenbal, J.-M. Gheller, A. Giganon, C. Lahonde-Hamdoun, D. Leboeuf, D. Loiseau, A. Mohamed, J.-Ph. Mols, H. Otsu, C. P´eron, A. Peyaud, E.C. Pollacco, G. Prono, J.-Y. Rousse, C. Santamaria, and T. Uesaka
- Nuclear Instruments and Methods in Physics Research A 840, 15 (2016)
J. Giovinazzoa, T. Goigouxa, S. Anvarc, P. Baronc, B. Blanka, E. Delagnesc, G.F. Grinyerb, J. Pancinb, J.L. Pedrozaa, J. Pibernata, E. Pollaccoc, A. Rebiia, T. Rogerb, P. Sizunc